Cell-to-cell interactions between melanocytes and keratinocytes increase the proliferation and migration of melanocytes. In fact, mixed keratinocyte and melanocyte cultures have been used for autologous cell transplantation for treatment of vitiligo. However, this may require taking an amount of skin tissue large enough to leave scars. In this study, the in vitro effect of adipose-derived stem cells (ADSCs) on proliferation, differentiation and migration of melanocytes was compared with that of keratinocytes using immunohistochemistry and a Boyden chamber migration assay. The proliferation and migration of melanocytes was significantly stimulated by co-culture with ADSCs compared with melanocyte monocultures, although the effect of ADSCs was less powerful than that of keratinocytes. This may be related to increases in stem cell factor and basic fibroblast growth factor, growth factors for melanocytes, produced by the ADSCs. The ratios of melanocytes stained with antibodies against Trp-2, E-cadherin and N-cadherin were significantly increased by co-culturing with ADSCs compared with co-culturing with keratinocytes as well as melanocyte monocultures. The proportion of less-pigmented melanocytes was also increased and sustained for a longer duration in the presence of ADSCs. Our data show that co-culturing with ADSCs results in increased melanocyte proliferation and migration while reducing differentiation, and could provide a means to treat disorders such as vitiligo. Key words: adipose-derived stem cells; keratinocytes; co-culture; melanocytes; proliferation; differentiation; migration.
(Accepted March 23, 2011.)
Acta Derm Venereol 2011; 91: XX–XX.
Ai-Young Lee, Department of Dermatology, Dongguk University Ilsan Hospital, 814 Siksa-dong, Ilsandong-gu, Goyang-si, Gyeonggi-do 410-773, Korea. E-mail: lay5604@naver.com
Vitiligo is a pigmentation disorder caused by the loss of melanocytes. Pigmentation results from melanocyte proliferation, melanogenesis, migration, and/or increases in dendricity (1, 2). In particular, melanocyte proliferation and/or migration from neighbouring normal epidermis or hair follicles is required for re-pigmentation in vitiligo patients. In fact, one of the most effective and safe therapeutic methods for treating vitiligo, narrow-band ultraviolet B radiation, stimulates cultured melanocyte proliferation and migration (3). However, normal human melanocytes rarely undergo mitosis (4, 5), and slow recovery of pigmentation with conventional medical therapies has led to the development of surgical treatment methods, including grafting cultured autologous melanocytes (6, 7). In addition to proliferation and migration, differentiation of melanocytes affects the clinical outcomes of vitiligo treatments. A greater number of immature melanocytes might result in a better clinical outcome. Although the phenotypic features of melanocytes at different stages of maturation have not been clearly defined, cells expressing c-kit and Trp-2 (dopachrome tautomerase) are considered to be melanocyte precursors in the migration staging area of the neural crest-derived melanocyte lineage (8) and in C57BL/6 mouse embryonic hair follicles (9). In human skin, cells that are both c-kit-positive and tyrosinase-related protein-1 (Trp-1)-negative have been proposed as precursor melanocytes (10), whereas Trp-1-positive cells are thought to be differentiated melanocytes (9, 10).
Cell-to-cell interactions play an important role in homeostasis and regeneration of adult tissues. Cross-talk of resident cells has been observed between keratinocytes and melanocytes. In fact, direct cell-to-cell contact stimulates in vitro proliferation of melanocytes, and growth factors produced by adjacent keratinocytes regulate the proliferation and differentiation of melanocytes (11, 12). Keratinocytes produce and release numerous synergistic mitogens in culture, such as basic fibroblast growth factor (bFGF), endothelins (ETs), stem cell factor (SCF), hepatocyte growth factor (HGF), nerve growth factor (NGF), granulocyte macrophage colony stimulating factor, leukaemia inhibitory factor, α-melanocyte stimulating hormone (α-MSH) and adrenocorticotrophic hormone (13). Keratinocyte-derived growth factors, such as NGF, ET-1, and bFGF also promote melanocyte migration (14–16).
Moreover, cadherins, calcium-dependent cell adhesion receptors involved in cell-to-cell interactions, have been implicated in cell migration (17). In fact, E-cadherin has been shown to contribute to adhesion between melanocytes and keratinocytes (18), whereas N-cadherin was found to be expressed on dermal melanocytes and facilitate contact with fibroblasts in the skin dermis following dorsolateral migration during neural crest cell development (19). Cadherins have also been implicated in melanocyte differentiation with N-cadherin serving as a key factor in melanoblastic cell lines (20).
The increase in proliferation and migration of melanocytes is expected in mixed keratinocyte and melanocyte cultures. However, it is difficult to obtain sufficient skin tissue for autologous cell transplantation without scar formation. If adipose tissue could be used in place of keratinocytes, sufficient amounts of tissue for transplantation could be obtained by liposuction through a small incision. Furthermore, adipose-derived stem cells (ADSCs) can be isolated and cultured from human adipose tissue (21, 22). Although cell-to-cell interactions between ADSCs and melanocytes have not been well-studied, ADSCs were shown to increase HGF secretion following exposure to bFGF or epidermal growth factor (EGF) (23). In this study, the effects of ADSCs on in vitro proliferation, differentiation, and migration of melanocytes were examined and compared with those of keratinocytes.
MATERIALS AND METHODS
Normal human epidermal melanocyte culture
Skin specimens obtained from repeat Caesarean section deliveries and circumcisions were used for the cultures. The epidermis was separated from the dermis after treatment with 2.4 U/ml of dispase (Roche, Mannheim, Germany) for 1 h. The epidermal sheets were treated with 0.05% trypsin for 10 min to produce a suspension of individual epidermal cells. The cells were suspended in Medium 254 (Cascade Biologics, Portland, OR, USA) supplemented with foetal bovine serum (FBS), bovine pituitary extract, bovine insulin, hydrocortisone, bFGF, bovine transferrin, heparin, and phorbol 12-myristate 13-acetate (Cascade Biologics). Taking FBS into account, the concentration was 0.5%. When the cells reached 80% confluence, they were detached from the flask and seeded into other culture flasks in preparation for an experimental procedure or to maintain and propagate the cell culture. For the subsequent experiments, the concentration of all supplements in Medium 254 was reduced to 20% (supplement-starved Medium 254), which contained 0.1% concentration, taking FBS into account.
Normal human epidermal keratinocyte culture
The same processes described in the previous section were performed to obtain a suspension of individual epidermal cells. However, instead of supplemented Medium 254, the cells were suspended in EpiLife Medium (Cascade Biologics) supplemented with bovine pituitary extract, bovine insulin, hydrocortisone, human EGF and bovine transferrin (Cascade Biologics). When the cells reached confluence, they were detached from the flask and seeded into other culture flasks.
Adipose-derived stem cell preparation
Human ADSCs were provided as a gift from Dr Byung-Rok Do of Hurim BioCell Inc. (Seoul, South Korea). The cells were cultured in low-glucose Dulbecco’s Modified Eagle’s Medium (DMEM; Gibco/BRL, Grand Island, NY, USA) supplemented with 10% FBS (Gibco/BRL), 100 U/ml penicillin (Gibco/BRL), and 0.1 mg/ml streptomycin (Gibco/BRL). ADSCs were treated with 5 μg/ml mitomycin C (MMC; Sigma Chemical Co., St Louis, MO, USA) for 5 h to inhibit cell proliferation. MMC-treated cells were detached from the flask and seeded into other culture dishes for preparation of feeder cells.
Co-culture of adipose-derived stem cells or keratinocytes with melanocyte culture
To examine the effect of ADSCs or keratinocytes (feeder cells) on melanocyte growth, the same number of MMC-treated ADSCs or keratinocytes (1.5 × 104 cells/ml) were seeded into a 6-well plate (NUNC, Roskilde, Denmark). Twenty-four hours after the seeding the feeder cells, the supernatant was removed, and then normal human melanocytes suspended in the supplement-starved Medium 254 were added into the plate at densities of 2.5 × 102, 5 × 102, and 2.5 × 103 cells/ml. The supplement-starved Medium 254 without feeder cells was used for a control. During 3 weeks of culture, media were changed every 3 days without performing any other procedures. The proliferation, differentiation and migration of melanocytes in the presence or absence of feeder cells were compared using immunohistochemistry and/or a Boyden chamber cell migration assay.
Immunohistochemistry
The cultured cells were fixed with a 4% paraformaldehyde solution, and sequentially pre-incubated with 0.2% Triton X-100 for 10 min, then 3% bovine serum albumin for 1 h. They were then incubated with anti-c-kit (1:50 dilution; mouse monoclonal; No. sc17806, Santa Cruz Biotechnology Inc., Santa Cruz, CA, USA), Trp-2 (1:200 dilution; goat polyclonal; No. sc10451, Santa Cruz Biotechnology Inc.), Trp-1 (1:200 dilution; goat polyclonal; No. sc10443, Santa Cruz Biotechnology Inc.), E-cadherin (1:200 dilution; rabbit monoclonal; No. 1702-s, Epitomics, Burlingame, CA, USA), or N-cadherin (1:200 dilution; rabbit monoclonal; No. 1934-1, Epitomics) antibodies overnight at 4ºC. Primary antibody binding was detected by incubation with Alexa fluor-labelled goat anti-mouse IgG (1:200 dilution; #594; No. A21203, Molecular Probes, Eugene, OR, USA), Alexa fluor-labelled donkey anti-goat IgG (1:200 dilution; #594; No. A11001, Molecular Probes) or Alexa fluor-labelled goat anti-rabbit IgG (1:200 dilution; #488; No. A11008, Molecular Probes) for 1 h. The stained cells were observed using a fluorescence microscope (Dp Manager 2.1; Olympus Optical Co., Tokyo, Japan). For quantitative analysis of the antibody-positive cells, ten random fields were photographed.
Boyden chamber cell migration assay
Nucleopore filters with an 8 μm pore size (Corning Inc., Corning, NY, USA) were coated with type I collagen. Human melanocytes (6 × 103 cells/ml) were added to the upper chamber and the lower chambers were filled with identical concentrations (1.2 × 104 cells/ml) of either MMC-treated ADSCs or keratinocytes. After 72 h incubation, the filters were stained with Hoechst, and the cells on the upper side of the insert were removed with a cotton swab. Nine randomly selected fields were photographed (DMI6000B, Leica Lasertechnic, Heidelberg, Germany), and the migrated cells were counted. The migration was expressed as a percentage of migrated cells in co-cultures with either ADSCs or keratinocytes per migrated cells in monocultures.
Western blot analysis
Western blot analysis was performed using supernatants as well as total cell extracts. For analysis of cell extracts, as previously described (24), ADSCs and keratinocytes were homogenized in ice-cold homogenization buffer containing 50 mM Tris-base (pH 7.5), 150 mM NaCl, 2 mM EDTA, 1% glycerol, 10 mM NaF, 10 mM Na pyrophosphate, and protease inhibitors (0.1 mM phenylmethylsulphonylfluoride, 5 mg/ml aprotinin, and 5 mg/ml leupeptin). For analysis of supernatant, volume of each supernatant was measured, followed by filtration through a 0.45 mm pore-sized membrane (Millipore, Bedford, MA, USA). The filtered supernatant was concentrated using an Amicon Ultra 15 centrifugal filter device (Millipore), with measurement of the final volume. Equal amounts of the extracted protein (30 µg) were resolved using 8–12.5% SDS-PAGE and transferred onto nitrocellulose membranes. After blocking with 5% non-fat dry milk in Tris-buffered saline (pH 7.6), the membranes were incubated overnight with antibodies against SCF (mouse monoclonal; No. sc13126, Santa Cruz Biotechnology Inc.), bFGF (mouse monoclonal; No. 610870, BD Biosciences, San Jose, CA, USA), and β-actin (mouse monoclonal; No. A5441, Sigma) diluted 1:1,000 in blocking solution at 4ºC. The membranes were then incubated with anti-mouse horseradish peroxidase-conjugated antibody (No. 31430, Pierce Biotechnology, Rockford, IL, USA) diluted 1:3000 in blocking solution at room temperature. The membranes were treated with an enhanced chemiluminescence solution (ECL kit; Amersham Life Sciences, Buckinghamshire, UK), and the signals were captured on an Image Reader (LAS-3,000; Fuji Photo Film, Tokyo, Japan).
Statistical analysis
Statistical significance was assessed with analysis of variance (ANOVA) tests. All results are presented as the mean ± standard deviation (SD) of the combined data from three or six independent experiments.
RESULTS
Stimulation of melanocyte proliferation by adipose-derived stem cells vs. keratinocytes
Before examining the effect of feeder cells on melanocyte proliferation, the proliferative activity of feeder cells in the supplement-starved Medium 254 was examined. Because ADSCs, but not keratinocytes, proliferated in the Medium 254, 5 μg/ml MMC was added to the medium to reduce cell proliferation by less than half during the 3-week culture period (data not shown). A fixed number of MMC-treated ADSCs were co-cultured with different quantities of melanocytes for 3 weeks.
The seeded number of melanocytes was less than 10% of feeder cells in co-cultures, and an MTT assay was not appropriate for discrimination of the numerical change in melanocytes (data not shown). Since ADSCs and keratinocytes were not stained with anti-c-kit antibody (Fig. 1A), the numerical change in melanocytes was examined by counting c-kit-positive cells. ADSCs significantly stimulated melanocyte proliferation, but to a lesser degree than keratinocytes (p < 0.005 vs. melanocytes; p < 0.05 or 0.005 vs. co-culture with keratinocytes) (Fig. 1). Although the difference in melanocyte proliferation between monoculture and co-culture was generally more pronounced with longer culture duration, ADSCs increased the number of melanocytes more at 2–3 weeks compared with 1–2 weeks, whereas co-culturing with keratinocytes increased the number of melanocytes more at 1–2 weeks (Fig. 1). Large numbers of keratinocytes, but not ADSCs, died at 3 weeks in the supplement-starved Medium 254. Similar differences were found in the range 2.5 × 102 to 2.5 × 103 cells/ml of melanocytes (data not shown).
Fig. 1. Results of 2.5 × 103 cells/ml of melanocytes (MCs) cultured with or without 1.5 × 104 cells/ml of adipose-derived stem cells (ADSCs) or keratinocytes (KCs) for 3 weeks. ADSCs were pretreated with 5 μg/ml mitomycin C (MMC), which was enough to reduce cell proliferation by 50% or more in the 3-week cultures. (A) Immunohistochemistry with anti-c-kit-stained MCs emitted a red fluorescence, whereas ADSCs and KCs did not (Scale bar =100 μm). (B) The number of c-kit-positive cells was counted in 10 random microscopic fields for statistical analysis. The data represent the mean ± SD of six independent experiments. The number of stained cells was markedly increased in co-cultures with both ADSCs (MCs+ADSCs) and KCs (MCs+KCs) over time. (*p < 0.005 vs. MCs, #p < 0.05 vs. MCs+KCs, ##p < 0.005 vs. MCs+KCs).
Adipose-derived stem cells induced more Trp-2 positive melanocytes
In addition to the effect on melanocyte proliferation, the effect of ADSCs on melanocyte differentiation was compared with that of keratinocytes. In fact, melanocytes were less-pigmented in the presence of ADSCs than melanocyte monocultures (Fig. 2A). The proportion of less-pigmented melanocytes was also increased in co-culturing with keratinocytes; however, they lasted for a shorter period of time compared with co-culturing with ADSCs (Fig. 2A). Expression of tyrosinase and Trp-1 showed correlation with melanin content and Trp-1 is positive only in differentiated melanocytes (9, 10). Trp-2 is expressed in both precursor and differentiated cells, showing a close association with regulation of cell growth and survival rather than differentiation of melanocytes (25). Therefore, the ratios of the cells stained with anti-Trp-1 or Trp-2 antibody to the ones stained with anti-c-kit antibody were examined. Although co-culturing with either ADSCs or keratinocytes significantly increased the number of melanocytes, the proportion of Trp-2-positive cells was significantly (p < 0.005) increased in the presence of ADSC feeder cells compared with melanocyte monocultures, and the proportion of Trp-1-positive cells was significantly (p < 0.005) reduced (Figs 2B and C). However, no difference was detected in the Trp-2-positive cells in the presence of keratinocyte feeder cells compared with monocultures, although the number of Trp-1-positive cells was significantly decreased (Figs 2B and C). Similar differences were found in 5 × 102 and 2.5 × 103 cells/ml melanocyte cultures (data not shown).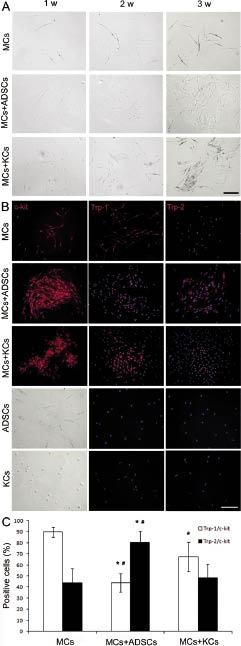
Adipose-derived stem cells increased expression of N-cadherin and E-cadherin in melanocytes
The melanocytes in adult human epidermis express E-cadherin (26), but not N-cadherin (27). N-cadherin has been reported to be the principle cadherin expressed by melanoblastic cell lines (20). Although keratinocytes were stained with anti-E-cadherin antibody (Fig. 3A) as reported (28), they could be discriminated from melanocytes based on the cell morphology. Moreover, the number of attached keratinocytes was reduced with passage of time, because melanocyte culture media could not provide proper conditions for keratinocyte growth. Melanocytes were stained with antibodies against these cadherins, whereas ADSCs were not (Fig. 3A). Therefore, expression of these cadherins in melanocytes was examined in the presence or absence of ADSCs or keratinocytes, by determining the ratio of cadherin-staining cells to c-kit-positive cells.
Fig. 3. Results of 2.5 × 103 cells/ml of melanocytes (MCs) cultured with or without 1.5 × 104 cells/ml of mitomycin C-treated adipose-derived stem cells (ADSCs) or keratinocytes (KCs) stained with antibodies against c-kit, E-cadherin, or N-cadherin. (A) The cells stained with c-kit had a red fluorescence signal, whereas those stained with E-cadherin or N-cadherin had a green fluorescence. ADSCs were not stained with any of the antibodies, although KCs stained with anti-E-cadherin antibody (scale bar = 100 μm) (B) The ratio of E-cadherin- or N-cadherin-positive cells to c-kit-positive cells was analysed. The number of stained cells was counted in 10 random microscopic fields. Data represent the mean ± SD of six independent experiments. Co-culture with ADSCs (MCs+ADSCs) increased both the E-cadherin- and N-cadherin-positive ratios; however, co-culture with KCs (MCs+KCs) increased only E-cadherin-positive ratios, compared with monoculture (MCs). (*p < 0.005 vs. MCs, #p < 0.005 vs. MCs+KCs).
The ratios of E-cadherin- and N-cadherin-positive melanocytes were significantly higher in the presence of the ADSCs (p < 0.005 vs. melanocytes; p < 0.005 vs. co-culture keratinocytes) (Fig. 3). However, the ratio of N-cadherin-positive melanocytes showed no significant change with the keratinocyte feeder, whereas that of E-cadherin-positive cells was increased (Fig. 3). Similar differences were found in the 5 × 102 and 2.5 × 103 cells/ml melanocyte cultures (data not shown).
Adipose-derived stem cells stimulated melanocyte migration less than keratinocytes did
The effect of keratinocytes on melanocyte migration has been documented (14–16) and was compared with that of ADSCs in our study. The Boyden chamber assay showed significantly (p < 0.005) increased cell migration of the melanocytes cultured in the presence of ADSCs compared with monocultures although the proportion of migrated melanocytes was lower than that observed in the presence of keratinocytes (Fig. 4).
Melanocyte growth factors produced by adipose-derived stem cells vs. keratinocytes
Although ADSCs have been shown to increase HGF secretion following exposure to bFGF or EGF (24), there is no report about ADSC production of the other growth factors for melanocytes including SCF, one of the most well-known growth factors for melanocytes (8, 29). Keratinocytes, on the other hand, are generally considered to be the main source of several growth factors for melanocytes including SCF (13). In fact, expression of bFGF was definite in the supernatant of keratinocytes (Fig. 5A). In the case of SCF, 23 kDa of soluble form was recognizable in the keratinocyte supernatant, and the 45 kDa membrane-bound form (29) was detected in the cell extract (Fig. 5B).
Here, the expression levels of SCF and bFGF from ADSCs were compared with those from keratinocytes in both supernatant and cell extract portions using Western blot analysis. In cell extracts, similar expression levels of SCF (membrane-bound) and significantly (p < 0.05 or p < 0.005) higher levels of bFGF were examined in ADSCs compared with keratinocytes (Fig. 5B). However, much lower, unrecognized levels of SCF (soluble) and bFGF were found in the supernatant of ADSCs (Fig. 5A). The culture conditions also influenced the expression levels of SCF and bFGF in ADSCs. In particular, treatment with MMC reduced expression of these growth factors in cell extracts (Fig. 5B), whereas it was increased in supernatant (Fig. 5A).
DISCUSSION
The role of keratinocytes in melanocyte proliferation is well-known (11, 12); however, the role of ADSCs has not been reported previously. The findings of this study showed that co-culturing with ADSCs increased the number of melanocytes more than monocultures (Fig. 1) although the effect was less significant and occurred more slowly than that of co-culturing with keratinocytes. In this study, the supplement-starved Medium 254, which contained 1/5 the amount of supplements, instead of the fully-supplemented culturing Medium 254 was used to maintain the viability, but not the growth, of melanocytes (30). The increase in proliferation was detected in melanocyte numbers as low as 5 × 102 cells/ml, almost the limit of growth in the monoculture (data not shown). In the co-culturing samples, a lower cell number and slower growth rate of melanocytes may result in lower optical densities than the actual cell number. Thus, the changes in cell number were detected consistently by using immunohistochemistry to monitor the c-kit-positive cell count (Fig. 1), but not by the MTT assay (data not shown). Reduced effects of keratinocytes as time goes by may result from the reduced number of cells that survived in the Medium 254 regardless of supplementation (data not shown), whereas more pronounced effects of ADSCs over time resulted from an increase in the ADSC cell number even with MMC pre-treatment. In addition to ADSC-induced HGF secretion (23), ADSCs produced SCF and bFGF (Fig. 5). However, ADSC-derived growth factors were not detected in the culture supernatant without MMC treatment, whereas keratinocyte-derived factors were released in the supernatant in the absence of MMC (Fig. 5), which suggested that ADSC-derived factors may differ from keratinocyte-derived ones. The difference may be responsible for lower rates of melanocyte proliferation in the presence of ADSCs compared with keratinocytes, although further studies including analysis of the amino acid sequence of the growth factor are required.
Melanocytes are present in the skin at different stages of maturation. It has been reported that Trp-1 is positive only in differentiated melanocytes, whereas Trp-2 is positive in precursor and differentiated cells. In this study, co-culturing with ADSCs significantly increased the proportion of Trp-2-positive melanocytes, not only compared with the melanocyte monoculture but also the keratinocytes co-culture (Fig. 2B and 2C). On the other hand, co-culturing with ADSCs significantly reduced the number of Trp-1-positive cells, similar to co-culturing with keratinocytes, compared with the melanocyte monoculture (Fig. 2B and 2C). Since expression of Trp-2 has not been associated with pigmentation, whereas Trp-1 shows correlation with melanin content (25), more Trp-2-positive cells and reduced Trp-1-positive cells in co-culturing with ADSCs (Fig. 2B and 2C) may support the result showing that the increased proportion of less-pigmented cells was sustained for a longer period of time in the presence of ADSCs than keratinocytes (Fig. 2A). Moreover, an increase in Trp-2-positive cells may relate to the increase in the total number of melanocytes in the co-culture with ADSCs, because expression of Trp-2 has also been shown to have a close association with regulation of cell growth and survival of melanocytes (25). Although the mechanism of the increased proportion of Trp-2-positive cells in co-culture with ADSCs was uncertain, one of the growth factors induced by ADSCs, bFGF (Fig. 5), may inhibit the differentiation of melanocytes (31). If more precursor melanocytes survived for longer, better clinical outcomes in treating diseases associated with melanocyte loss could be obtained with lower numbers of transplanted melanocytes. Cadherins are transmembrane glycoproteins connected to the actin cytoskeleton, and mediate haemophilic adhesion between neighbouring cells. Cell-to-cell adhesion via cadherins has been implicated in morphogenesis (32). Since N-cadherin expression has been found in melanoblastic cell lines (20), but not in melanocytes (27), the increase in N-cadherin-positive melanocyte ratios in co-cultures with ADSCs compared with the keratinocyte co-cultures as well as monocultures (Fig. 3A and 3B) may also indicate that co-culturing with ADSCs increased the number of melanocyte precursor cells.
Fig. 5. Western blot analysis with anti-stem cell factor (SCF) and anti-basic fibroblast growth factor (bFGF) antibodies was performed in both supernatant and cell extract fractions. Adipose-derived stem cells (ADSCs) pretreated with or without 5 μg/ml mitomycin C (MMC) were cultured in the supplement-starved Medium 254 (MC medium) for 2 days. ADSCs cultured in the original medium without pretreatment (ADSC medium) for 2 days are also shown. KCs were cultured in the original medium (KC medium) and MC medium for 2 days. Data represent the mean ± SD of three independent experiments. (A) Expression of bFGF and SCF (23 kDa, soluble form) in supernatants released from cultured keratinocytes (KCs) and ADSCs could be estimated by extracting the expression level in each medium without cultured cells (Control). In KCs, these growth factors were detected in supernatants; however, these were recognized in ADSC supernatants with MMC treatment. (*p < 0.05) (B) Expression of bFGF and SCF (45 kDa, membrane-bound form) in cell extracts of KCs and ADSCs cultured under different conditions. Similar expression levels of SCF and higher levels of bFGF were examined in ADSCs compared with KCs. For the recognizable expression of bFGF in KCs, the blotted membrane was exposed for 10 times longer (*p < 0.05, **p < 0.005).
However, the expression patterns of cadherins and tyrosine-related proteins are complicated. Different degrees of maturation could occur in the differentiated and precursor cells, as shown by Trp-2 positive and Trp-2/Trp-1 double-positive melanocytes in C57BL mouse hair follicles (9). It is more likely that the changes in cadherin expression suggest that co-culturing with ADSCs affects cell migration (33). Melanocyte precursor cells from the neural crest pass through the dermis and then to the epidermal basal layer and hair follicles (34). N-cadherin plays a role in adhesion of dermal melanocytes to fibroblasts during the course of migration (19), whereas E-cadherin plays a role in adhesion of epidermal melanocytes to keratinocytes (19, 35), therefore, the melanocyte precursor cells could produce E-cadherin on their cell surface during migration prior to entry into the epidermis (17). Co-culturing with ADSCs not only induced more N-cadherin expression, but also more E-cadherin expression, compared with keratinocyte co-cultures. However, the Boyden chamber migration assay showed that co-culturing with ADSCs stimulated melanocyte migration less than co-culturing with keratinocytes, although both cell types significantly stimulated migration compared with melanocyte monocultures (Fig. 4). In fact, the keratinocyte-derived growth factors, such as NGF, ET-1, and bFGF, promote melanocyte migration (14–16) and further studies are required to identify the mechanism.
Fig. 4. The Boyden chamber cell migration assay was performed using melanocytes (MCs; 6 × 103 cells/ml) in the upper chamber and mitomycin C-treated adipose-derived stem cells (ADSCs) (1.2×104 cells/ml) or keratinocytes (KCs) (1.2 × 104 cells/ml) in the lower chamber. (A) After 72 h incubation, the nuclei were stained with Hoechst stain. (Scale bar = 100 μm). (B) The ratio of Hoechst-stained migrated cells to control MCs was analysed. The number of stained nuclei was counted in 9 random microscopic fields. Data represent the mean ± SD of six independent experiments. Compared with monoculture (MCs), co-culturing with ADSCs (MCs+ADSCs) increased the ratio of migrated MCs, although this ratio was less than one for co-culture with KCs (MCs+KCs) (*p < 0.005).
In summary, co-culturing with ADSCs stimulated both proliferation and migration of melanocytes although this was to a lesser degree compared with co-culturing with keratinocytes. ADSC co-culturing also increased the number of melanocyte precursor cells. These findings may be used to improve treatments of pigmentation disorders associated with the loss of melanocytes.
ACKNOWLEDGEMENTS
This study was supported by a grant from the Korea Healthcare Technology R&D Project (A080980), Ministry of Health Welfare and Family Affairs, Republic of Korea.
REFERENCES